Advanced Bio-Mechanical Performance of Additively Manufactured Bone Scaffolds Using Beta Ti Alloys and Gyroid-Based Cellular Structures
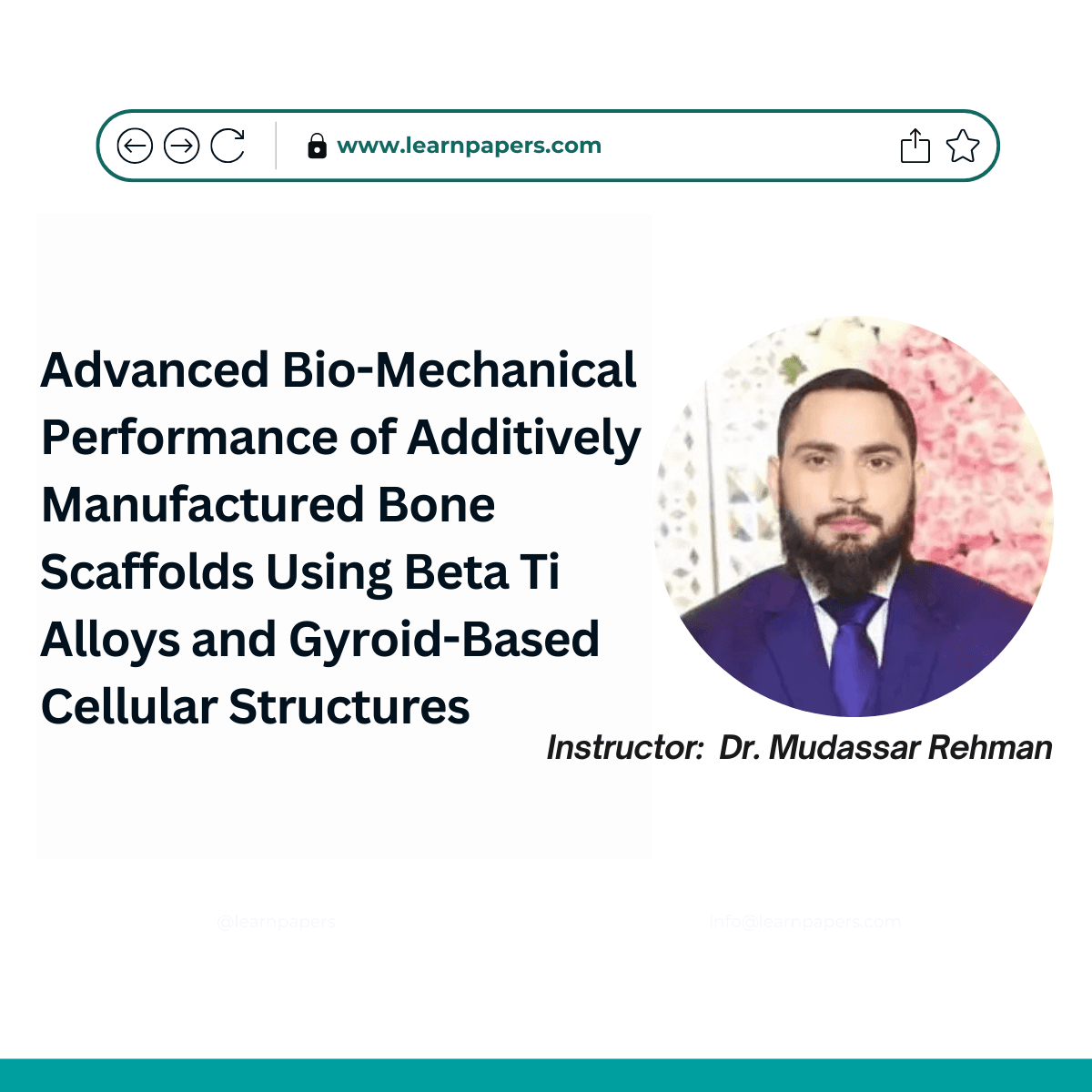
About Course
Biomedical implants are transforming the future of healthcare, and advancements in additive manufacturing (3D printing) are making patient-specific orthopedic solutions more effective than ever. This course explores the biomechanical properties, fabrication techniques, and performance analysis of beta titanium (β-Ti) alloys, which are revolutionizing medical implant design. By leveraging Laser Powder Bed Fusion (L-PBF), researchers and engineers can optimize bone scaffolds with superior strength, biocompatibility, and durability.
Through this course, learners will gain a comprehensive understanding of the interplay between material composition, manufacturing processes, and clinical applications of β-Ti alloys in orthopedic implants. By the end of the program, participants will be able to analyze scaffold performance, optimize implant designs, and apply biomechanical principles to create advanced bone-regenerating implants that enhance patient outcomes.
Abstract:
An exponential rise in demand for artificial implants has emphasized the need for novel biomaterials with good biocompatibility and mechanical properties comparable to human cortical and cancellous bone, to mitigate stress shielding and other post-implantation challenges. This study investigated the processing performance of biomedical β-Ti alloys (Ti-12Ta-23Nb-24Zr, Ti-32Nb-25Zr) fabricated via Laser Powder Bed Fusion (L-PBF) under optimal working conditions. These processing conditions included laser power (200 W), scanning speed (1000 mm/s), hatch distance (0.065 mm), layer thickness (40 μm), and exposure time (50 μs). It was found that Ti-12Ta-23Nb-24Zr exhibited the highest compressive strength (864 MPa), near bone elastic modulus (45.2 GPa), higher bone regrowth rate (111.6%), minimum process-induced porosity (3.681%), and reduced surface roughness (0.462 μm), making it ideal for orthopedic implants. On the other hand, Ti-32Nb-25Zr also demonstrated good compressive strength (842 MPa), near bone elastic modulus (53 GPa), minimum process-induced porosity (4.285%), and good bone regrowth rate (94.7%), suitable for initial bone integration. It was found that beta Ti alloys have improved bio-mechanical performance compared to conventional Ti, in the order Ti-12Ta-23Nb-24Zr > Ti-32Nb-25Zr > Ti-6Al-4V. The findings underscore the adaptability of optimized L-PBF settings across biomedical β-Ti alloys, paving the way for advanced patient-specific orthopedic implants.